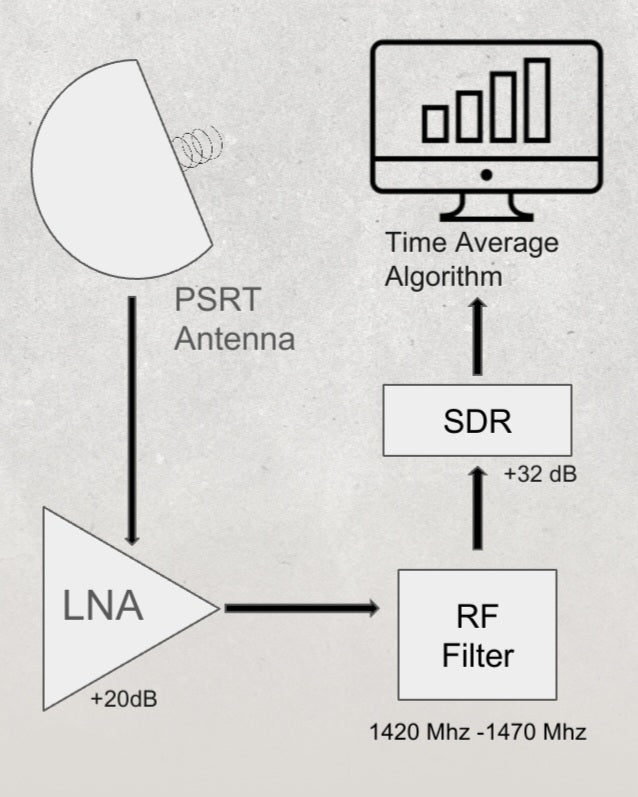
Pulsar-Sensitive Radio Telescope (PSRT) Receiving System Overview
Diagram of the PSRT receiving system, showcasing the signal path from antenna capture to digital processing, with components optimized for high-sensitivity radio astronomy in the 1420–1470 MHz range.
The receiving system diagram for the PSRT demonstrates a meticulously engineered signal path optimized for high-sensitivity radio astronomical observations, specifically within the hydrogen line frequency range of 1420–1470 MHz. This configuration incorporates several critical components in sequence, each with specialized functions to amplify, filter, and process incoming radio signals to ensure precise data capture and analysis.
1. PSRT Antenna
The system begins with the PSRT antenna, a highly sensitive dish structure designed to collect faint radio emissions from celestial sources. This antenna is likely configured as a parabolic reflector, a geometry commonly used in radio astronomy due to its ability to focus incoming radio waves onto a single feed point with minimal loss. This focal concentration maximizes the gain and improves signal-to-noise ratio (SNR), crucial for detecting weak astronomical signals amidst background noise.
The antenna operates within the 1420–1470 MHz range, which covers the hydrogen line (1420 MHz), a significant frequency in radio astronomy used for observing neutral hydrogen in the interstellar medium. The reception of signals within this band allows for the study of galactic and extragalactic hydrogen clouds, key to understanding the structure and evolution of the cosmos.
2. Low-Noise Amplifier (LNA)
After initial capture, the signal is fed into a Low-Noise Amplifier (LNA) stage. The LNA is specified to provide a gain of +20 dB, which serves to amplify weak radio frequency (RF) signals while minimizing additional noise. In radio astronomy, LNAs are chosen for their ultra-low noise figures to preserve the integrity of weak astronomical signals. The +20 dB gain ensures that even minimal signals are amplified sufficiently before proceeding to the next stage, enhancing the sensitivity of the overall system.
By amplifying the signal at this stage, the LNA helps to mitigate the effect of any subsequent noise added by downstream components. This setup is especially critical for faint signals where noise in later stages could overwhelm the signal if not amplified early.
3. RF Filter
Following amplification, the signal passes through an RF (Radio Frequency) filter. This filter is finely tuned to the target frequency range of 1420–1470 MHz, effectively eliminating out-of-band frequencies that could interfere with or degrade the quality of the desired signal. By isolating this narrow band, the RF filter ensures that only signals within the astronomical hydrogen line range reach the subsequent processing stages.
The selectivity of this RF filter is crucial for rejecting unwanted interference from terrestrial sources, such as telecommunications signals, that fall outside the designated passband. This approach maintains the purity of the data and allows the PSRT to focus solely on capturing astrophysical signals within the specified range.
4. Software-Defined Radio (SDR)
The filtered and amplified signal then enters a Software-Defined Radio (SDR) module, which provides an additional gain of +32 dB. The SDR is a versatile, reconfigurable digital receiver that digitizes the analog signal and processes it using software algorithms. The +32 dB gain applied at this stage further enhances the signal’s strength, preparing it for in-depth digital processing.
The SDR is integral to the flexibility of the PSRT system, as it allows for dynamic adjustments in frequency, bandwidth, and modulation analysis through software. This adaptability makes the PSRT capable of real-time adjustments based on observational requirements or interference conditions, ensuring optimal performance across various observation scenarios.
5. Time-Averaging Algorithm
Once digitized, the signal data undergoes processing through a time-averaging algorithm, which is applied on a computer or data processing unit. This algorithm serves two main purposes:
Noise Reduction: By averaging the signal over time, random noise fluctuations are minimized, enhancing the SNR of the detected astronomical signals. This is particularly important in radio astronomy, where signals are inherently weak and often masked by thermal noise.
Data Integrity: Time-averaging provides a smoother, more coherent representation of the signal, allowing astronomers to discern subtle patterns in the data that may be indicative of astrophysical phenomena. This algorithm aids in isolating persistent signals from transient noise, facilitating more accurate detection of hydrogen emissions or other targeted astronomical sources.
The time-averaging process is key to refining the data for subsequent scientific analysis, enhancing the PSRT’s ability to detect faint cosmic signals with high precision.